Unveiling the Impact of Critical Strain in Materials Engineering
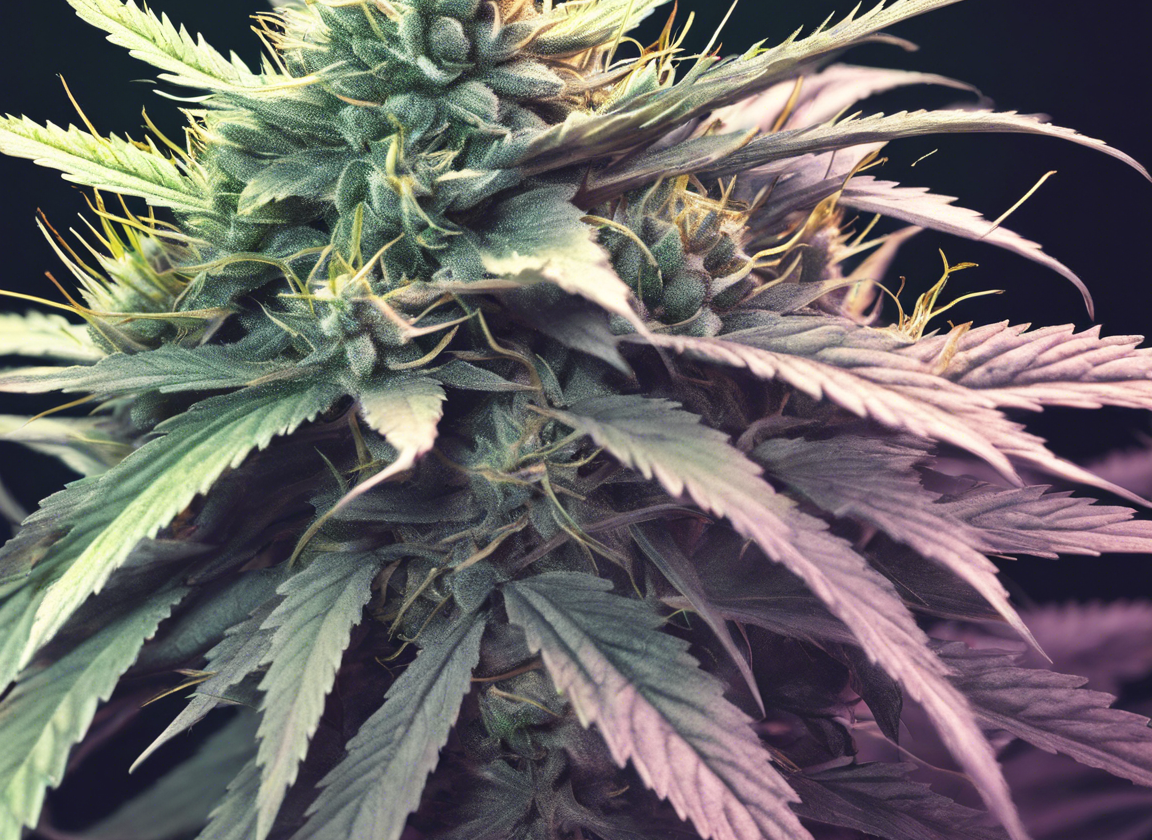
Before delving into the impact of critical strain in materials engineering, it is essential to understand the concept itself. Critical strain is a crucial parameter that dictates the mechanical behavior and failure mechanisms of materials under applied loads. In simple terms, it is the maximum amount of deformation a material can undergo before experiencing failure or breakage. This article will explore the significance of critical strain in various types of materials, its measurement methods, and its practical implications in the field of materials engineering.
Understanding Critical Strain
Critical strain is a fundamental property of materials that characterizes their ability to deform elastically and plastically under stress. In engineering terms, it represents the point at which a material transitions from elastic deformation (reversible deformation under load) to plastic deformation (permanent deformation). Beyond the critical strain, the material may undergo necking, fracture, or other forms of failure.
Types of Critical Strain
There are two primary types of critical strain that engineers consider:
- Yield Strain: This is the strain at which a material begins to deform plastically.
- Fracture Strain: This is the strain at which a material fails under applied stress.
Importance of Critical Strain in Materials Engineering
Design Considerations
Understanding the critical strain of materials is crucial in the design process as it directly impacts the selection of materials for specific applications. Engineers need to know the yield strain of a material to ensure that it can withstand the expected loads without deforming permanently. Similarly, knowledge of the fracture strain helps in designing structures and components that can tolerate sudden stress spikes without catastrophic failure.
Material Selection
The critical strain of a material influences its suitability for different engineering applications. For example, materials with high yield strain are preferred in structural components where deformation needs to be limited, such as in buildings or bridges. On the other hand, materials with high fracture strain are chosen for applications where toughness and ductility are critical, like in automotive crash zones.
Failure Prediction
By determining the critical strain of materials through advanced testing and analysis, engineers can predict and prevent potential failure scenarios in structures and machinery. This proactive approach helps in enhancing the safety and reliability of engineered systems, thereby avoiding costly downtime and repairs.
Measurement of Critical Strain
Tensile Testing
One of the most common methods for measuring critical strain is through tensile testing. In this test, a sample of the material is subjected to increasing tensile load until it reaches its breaking point. By analyzing the stress-strain curve obtained from the test, engineers can identify the yield point and the fracture point, thus determining the critical strain values.
Finite Element Analysis (FEA)
Finite Element Analysis is another technique used to predict critical strain values in materials without the need for physical testing. By creating a digital model of the material and subjecting it to virtual loads, engineers can simulate the behavior of the material under different conditions and accurately predict the critical strain for specific applications.
Other Advanced Testing Methods
Apart from tensile testing and FEA, there are several advanced testing methods such as X-ray diffraction, acoustic emission testing, and digital image correlation that can be used to measure critical strain in materials with high precision and accuracy.
Practical Implications of Critical Strain in Materials Engineering
Aerospace Industry
In the aerospace industry, where lightweight materials with high strength are vital, understanding the critical strain of composites and alloys is crucial. By optimizing the critical strain values of materials used in aircraft components, engineers can enhance the structural integrity and performance of airplanes while reducing weight and fuel consumption.
Automotive Sector
In the automotive sector, materials with high fracture strain are preferred for manufacturing components like crumple zones and safety cages. These components are designed to absorb impact energy during collisions and protect the occupants of the vehicle. By selecting materials with the right critical strain properties, automakers can improve the overall safety standards of their vehicles.
Civil Engineering
In civil engineering, the critical strain of construction materials is essential for ensuring the durability and stability of buildings, bridges, and infrastructure projects. By estimating the critical strain values of concrete, steel, and other construction materials, engineers can design structures that can withstand environmental factors, seismic activities, and other external loads.
Frequently Asked Questions (FAQs)
1. How is critical strain different from critical stress in materials engineering?
Critical strain refers to the maximum deformation a material can undergo before failure, while critical stress is the maximum load a material can bear before failure. They are related parameters but represent different aspects of material behavior under loading conditions.
2. Can critical strain be altered or improved in materials through processing or treatment?
Yes, critical strain can be influenced by factors like alloying, heat treatment, cold working, and microstructural modifications. These methods can enhance the mechanical properties of materials and improve their critical strain values.
3. What is the role of temperature in determining critical strain in materials?
Temperature plays a significant role in affecting the critical strain of materials, especially in high-temperature applications. Elevated temperatures can reduce the critical strain values of materials, making them more susceptible to deformation and failure.
4. How do defects and imperfections in materials impact their critical strain values?
Defects like voids, inclusions, and dislocations can act as stress concentrators and reduce the critical strain of materials. It is essential to minimize defects during material processing to enhance their mechanical properties and critical strain values.
5. Is there a correlation between critical strain and material ductility?
Yes, there is a direct correlation between critical strain and material ductility. Materials with high critical strain values tend to exhibit greater ductility, allowing them to deform plastically before failure and absorb more energy during loading.
In conclusion, understanding the concept of critical strain is paramount for materials engineers seeking to optimize the mechanical performance and reliability of various materials in diverse applications. By accurately measuring critical strain values, selecting appropriate materials, and employing advanced testing methods, engineers can design structures and components that meet stringent safety and performance requirements in the ever-evolving field of materials engineering.